Translate this page into:
Evaluation of knee articular cartilage through calcium-suppressed technique in dual-energy computed tomography
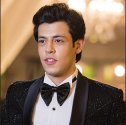
*Corresponding author: Vibhor Dhingra, Department of Radio-Diagnosis, Era’s Lucknow Medical College and Hospital, Lucknow, Uttar Pradesh, India. vibhor.dhingra@outlook.com
-
Received: ,
Accepted: ,
How to cite this article: Khanduri S, Siddiqui SA, Dhingra V, Imam TA, Jaiswal V, Bansal T, et at. Evaluation of knee articular cartilage through calcium-suppressed technique in dual-energy computed tomography. Indian J Musculoskelet Radiol. 2024;6:95-103. doi: 10.25259/IJMSR_8_2024
Abstract
Objectives:
The evaluation of knee articular cartilage is of paramount importance in diagnosing and managing musculoskeletal disorders. Accurate and non-invasive imaging techniques are essential for assessing cartilage health, guiding treatment decisions, and monitoring disease progression. The objective of this study is to assess the potential of a calcium-suppressed technique in dual-energy computed tomography (DECT) as a viable alternative to the gold standard magnetic resonance imaging (MRI) for the evaluation of knee articular cartilage. DECT is gaining momentum in musculoskeletal imaging due to its ability to differentiate tissues with high precision. By suppressing calcium signals in DECT, the cartilage can be visualized more effectively, providing valuable insights into its integrity and structural changes. This paper seeks to compare the diagnostic accuracy and overall performance of DECT with calcium suppression to conventional MRI. The findings of this research have the potential to revolutionize the way that we approach knee cartilage evaluation, making it more accessible and efficient for both clinicians and patients. This paper contributes to the ongoing efforts in advancing medical imaging and orthopedic diagnostics, ultimately improving patient outcomes and healthcare practices.
Material and Methods:
In this study, we included patients who had reported knee pain and were referred for both DECT and MRI examinations. To enhance cartilage visualization, calcium-suppressed images were generated through a specialized algorithm applied to spectral-based images and were then superimposed onto conventional computed tomography (CT) images for visual assessment. The structure and thickness of knee cartilage were meticulously examined, and measurements of articular cartilage thickness were taken from sagittal proton density-weighted images and calcium-suppressed images in at lateral femoral tibial, patella femoral, and medial femoral tibial region of knee.
Results:
The present study was done to compare the two diagnostic modalities (DECT and MRI) for assessment of cartilage thickness of patients with knee pain. Cartilage thickness was assessed at lateral femoral tibial, patella femoral, and medial femoral tibial region of knee. Cartilage thickness of only affected knee was assessed by DECT and MRI, which showed almost perfect agreement (differences non-significant) at all the regions: (Lateral femoral region 1.61 ± 0.55 mm vs. 1.62 ± 0.55; Patella femoral: 1.62 ± 0.70 vs. 1.65 ± 0.70 mm and Medial femoral tibial: 1.82 ± 0.78 vs. 1.86 ± 0.74 mm).
Conclusion:
This study showed that measurements of knee cartilage thickness by DECT were as reliable as that by MRI. This study compares DECT and MRI for assessing knee articular cartilage. The rationale for combining CT and MRI provides a comprehensive evaluation of bone and soft-tissue pathology, complex injuries, and pre-surgical planning. DECT excels in bone detail, while MRI excels in soft-tissue evaluation. Our findings suggest that DECT may replace MRI for cartilage thickness assessment and qualitative evaluation of knee abnormalities, offering a cost-effective alternative with improved accessibility and reduced contraindications. DECT benefits patients of knee pain with limited MRI access or with contraindications.
Keywords
Knee cartilage
Dual-energy computed tomography
Calcium suppression technique
Knee pain
Cartilage thickness
Magnetic resonance imaging
INTRODUCTION
Knee joint pain is a common issue, particularly among the elderly population. This musculoskeletal problem, if left unaddressed, can progress to more severe conditions like osteoarthritis (OA), potentially leading to physical disability in the elderly.[1] Knee pain not only impairs locomotor function but also contributes to reduced walking speed, decreased work productivity, and a decline in the overall quality of life.[2]
Studies have estimated that lower limb musculoskeletal pain affects a substantial portion of the adult population, with prevalence rates ranging from 20% to 32%. Additional risk factors for knee pain include being overweight, smoking, and a history of previous knee injuries.[3] Obesity, in particular, is a prominent risk factor, as it has been significantly associated with an increased risk of knee OA, a condition that becomes more prevalent with age and obesity.[4] Hypertension, diabetes, and obesity increase OA risk through mechanisms such as joint loading, microvascular damage, inflammation, glycation, oxidative stress, and metabolic dysregulation.
The aging population and rising rates of obesity have contributed to a substantial increase in the demand for total knee replacement procedures. The degeneration of cartilage between knee joints can lead to bone-on-bone contact, resulting in pain, reduced joint mobility, and swelling.[5] Clinical and radiological assessments of patients with knee pain are often conducted using the Kellgren–Lawrence (KL) grading system, which is based on weight-bearing anteroposterior radiographs of both knees. Higher KL grades indicate more severe signs of OA, often leading to surgical intervention, while lower grades may suggest physical therapy, yoga, and lifestyle modifications.[6]
Articular cartilage, a critical component in the knee joint, comprises approximately 80% water, along with cellular substances, intercellular matrix, and ground substance, giving it its characteristic firmness. It consists of four distinct zones, each with specific functions, providing a smooth and lubricated surface for joint articulation and load transmission[Figure 1].[7,8]
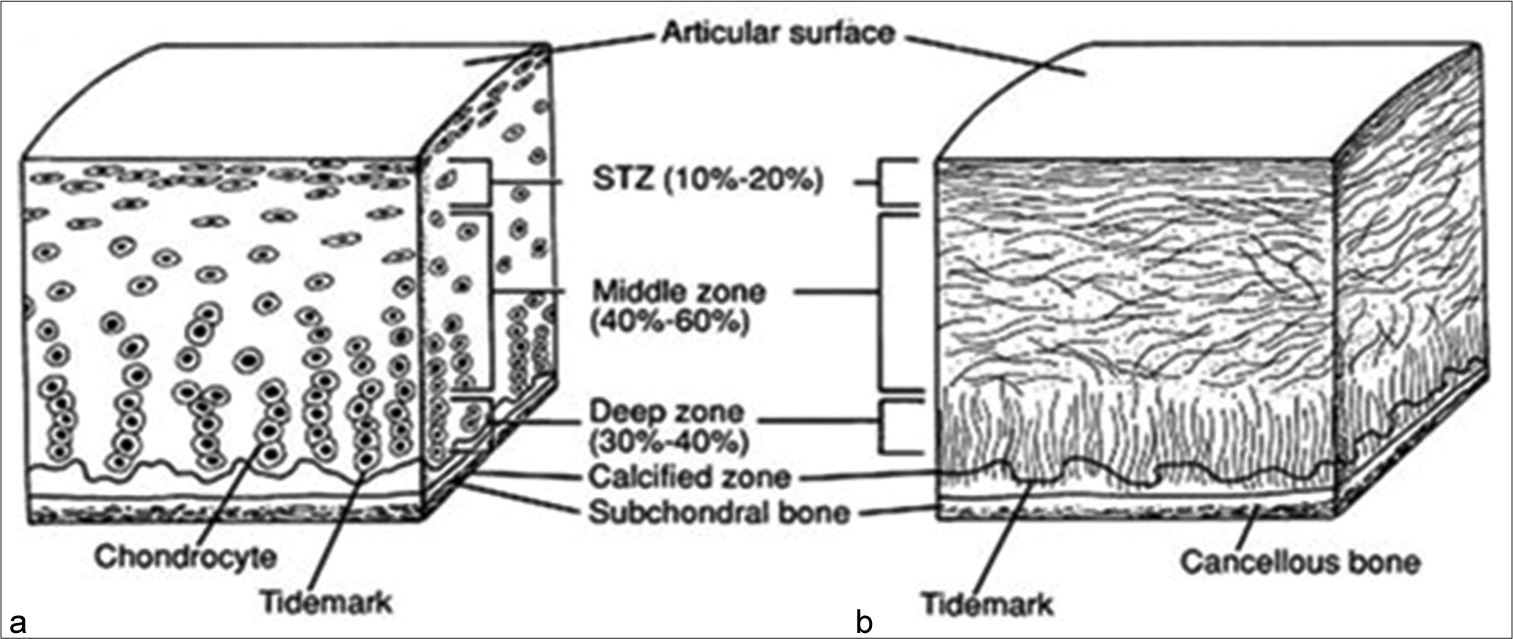
- Structure of articular cartilage. (a) Histologic section of cartilage from a young, healthy adult shows even safranin O staining and distribution of chondrocytes. (b) Schematic diagram of chondrocyte organization. Adapted from: Buckwalter et al. (1994).
When this cartilage undergoes degeneration, often due to factors such as long-term weight-bearing, inflammation, or degeneration, it can lead to joint damage, increased pain, and potential immobility.[9] One of the challenges in assessing cartilage damage is that it is not directly visible through traditional radiographs, which are primarily designed to detect bone abnormalities.[10]
While traditional ultrasonography provides limited insight into cartilage structure due to insufficient resolution, advanced ultrasonography with higher frequency probes can penetrate deeper, enabling the detection of additional information from articular cartilage, such as backscatter patterns.[11]
Magnetic resonance imaging (MRI) has emerged as a critical tool for diagnosing articular cartilage lesions, offering high-resolution, multi-parameter, multi-planar images with superior soft-tissue contrast, and without ionizing radiation.[12] However, MRI is primarily accessible in high-resource facilities.
Dual-energy computed tomography (DECT), an advanced computed tomography (CT) system utilizing two x-ray sources and data acquisition systems,[13] offers advantages over conventional CT, including superior soft-tissue resolution in spectral imaging and material-specific color mapping.[14] One of the applications of DECT is calcium suppression, a technique that enhances the assessment of pathological conditions, often improving on standard CT evaluations.[15]
The present study was conducted to evaluate the utility of DECT, particularly its calcium suppression technique, in assessing articular cartilage insults, with reference to MRI findings. This research seeks to determine whether DECT can be a valuable tool for non-invasive imaging of articular cartilage and potentially offer an alternative to traditional MRI in the assessment of knee joint conditions.
MATERIAL AND METHODS
The study design for this research was a comparative observational study conducted over a period of 24 months at the tertiary care hospital in the Department of Radiodiagnosis in collaboration with the Orthopedics Department. The study’s sampling frame consisted of patients who complained of knee pain and were referred to the Department of Radiodiagnosis for MRI and DECT examinations. Inclusion criteria were patients of both sexes aged 40 years or older with knee pain referred for these specific imaging tests. Pregnant females and patients with contraindications for MRI, such as those with ferromagnetic pacemaker implant or cochlear implants, were excluded from the study.
All eligible patients underwent clinical evaluation in the Department of Orthopedics. After obtaining informed consent, demographic information and side of knee involved, the extent of impairment was recorded. Subsequently, patients underwent imaging evaluation using both MRI and DECT.
The study received clearance and approval from the Institutional Ethical Committee at the tertiary care Hospital vide approval letter numbered R cell EC/2021/32, and informed consent was obtained from all participating patients.
Sample size was calculated on the basis of number of BML among study cases using the formula:
Where P = 75.0% the proportion of BML among study cases (Muratovic et al.[12])
q = 100 – p,
Type I error α = 5%, for the significance level of 95%.
Allowable error L = 15% absolute for detecting the results with 80% power of study,
Data loss = 10%
The minimum sample size required comes out to be n = 35.
Due to the novelty of DECT in knee cartilage assessment, we conducted a study with a small sample size (n = 35) to establish feasibility and optimize imaging protocols. Economic constraints resulted in a smaller, self-selected sample of patients able to undergo both DECT and MRI. This limitation may introduce selection bias, potentially affecting the generalizability of our findings. However, we believe that our study contributes to the understanding of knee articular cartilage assessment using DECT and MRI, and we recommend future studies address this economic barrier to improve representation. Moreover, this study focused on proof-of-concept for DECT/MRI comparison, and the small sample size (n = 35) was sufficient for hypothesis generation. This study serves as a preliminary investigation to determine feasibility and establish protocols for future larger-scale studies.
Patients’ clinical details, including the duration of pain, were recorded, and they underwent DECT and MRI examinations. DECT scans were performed on a 384-slice DECT scanner and interpreted by a radiologist. MRI examinations were conducted using a 3T MRI system and evaluated based on specific imaging sequences. The effective radiation dose in a DECT given to the patient was approximately 0.5–2.0 mSv. All images were analyzed retrospectively on a work station using Syngovia software, that allowed for analysis of images using calcium suppression technique in DECT, three-dimensional reconstructions and measurements.
Statistical analysis was performed using the Statistical Package for the Social Sciences software, and the results were represented using number and percentage as well as mean ± standard deviation. The statistical tools employed included Chi-square tests for categorical data comparison and paired “t” tests for cartilage thickness comparison, with a significance level of P < 0.05 considered statistically significant.
RESULTS
The age range of the patients participating in the study varied from 40 to 57 years, with an average age of 47.26 years, showing that the majority of patients were older than 45 years.
Age of patients enrolled in the study ranged between 40 and 57 years. Mean age of patients was 47.26 ± 4.82 years. Majority of the patients were aged >45 years. The study population was predominantly male, accounting for 62.9% of the participants, resulting in a gender ratio of 1.69.
The duration of severe knee pain that required attention from a physician ranged from 1 day to 90 days, with an average duration of 19.91 days. More than half of the patients (51.4%) had experienced knee pain for over 15 days, and 11.4% had knee pain persisting for more than 30 days.
Among the 35 patients with knee pain in the study, 22.9% had a history of knee injury, and 31.4% reported a family history of OA. The study also found that 25.7% of the participants had hypertension, 37.1% had diabetes mellitus, and 34.3% were obese. Hypertension causes increased joint loading, microvascular damage, and inflammation and increases the risk of OA. Diabetes mellitus causes advanced glycation end-products, oxidative stress, and cartilage damage. Obesity causes mechanical loading, inflammation, and metabolic dysregulation. Obesity is a strong risk factor for OA, especially in knee joints.
Cartilage thickness measurements, obtained using both DECT and MRI, were quite similar.
Patellofemoral cartilage thickness measured 1.65 ± 0.70 mm with MRI [Figure 2a] and 1.62 ± 0.70 mm with DECT [Figure 2b].
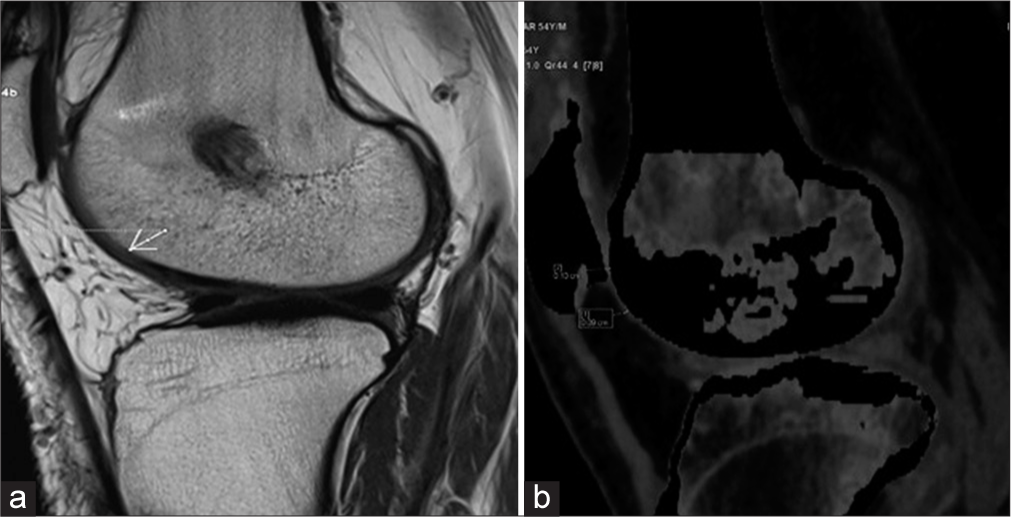
- (a) Proton density sagittal magnetic resonance image showing the patellofemoral cartilage. (b) Sagittal dual-energy computed tomography with calcium suppression technique image shows patellofemoral cartilage. The arrow indicates Areas of cartilage thinning is consistent with degenerative changes observed in osteoarthritic conditions.
Lateral femoral tibial cartilage thickness, for instance, measured 1.62 ± 0.55 mm with MRI [Figure 3a] and 1.61 ± 0.55 mm with DECT [Figure 3b].
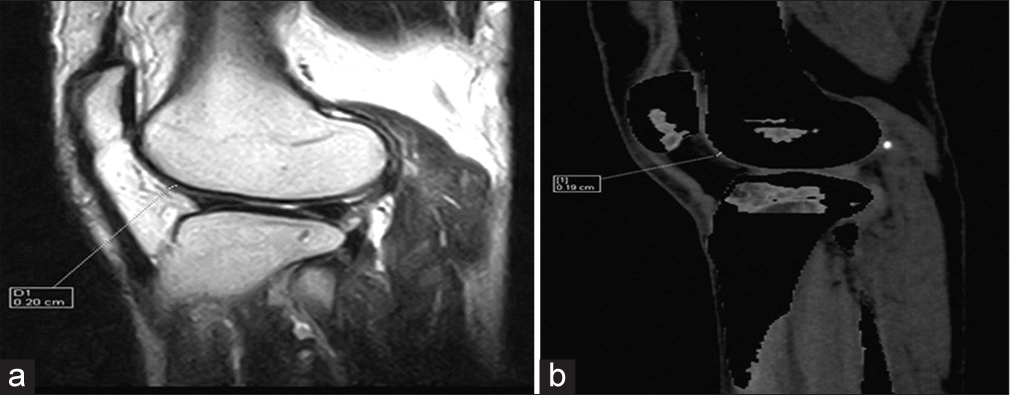
- (a) Proton density sagittal magnetic resonance image shows lateral femoral tibial cartilage thickness. (b) Sagittal dual-energy computed tomography with calcium suppression technique image shows lateral femoral tibial cartilage thickness.
Similarly, medial femoral tibial cartilage thickness was 1.86 ± 0.74 mm with MRI [Figure 4a] and 1.82 ± 0.78 mm with DECT [Figure 4b].
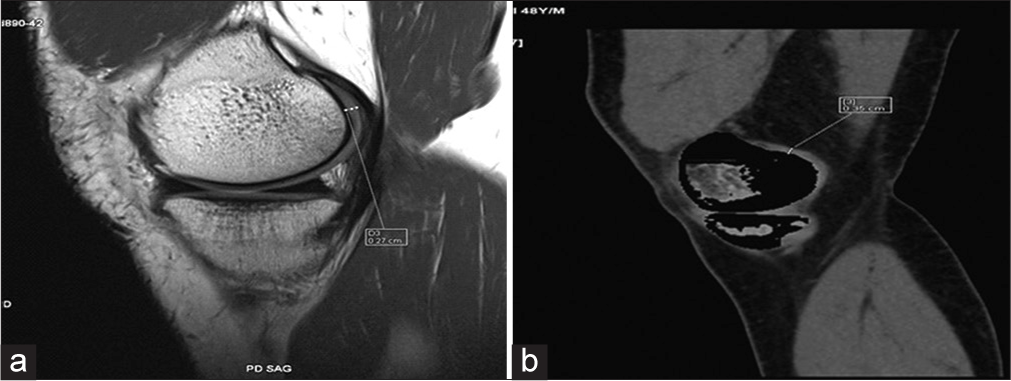
- (a) Proton density sagittal magnetic resonance image shows medial femoral tibial cartilage thickness. (b) Sagittal dual-energy computed tomography with calcium suppression technique image shows medial femoral tibial cartilage thickness.
The study found no statistically significant differences [Table 1 and Figure 5] in cartilage thickness measurements between DECT and MRI based on factors such as age, gender, duration of pain, and comorbidities [Tables 2 and 3], except for medial femoral tibial cartilage thickness and obesity, which showed a significant association [Table 4 and Figure 6].
CT findings | MRI Findings | |||||
---|---|---|---|---|---|---|
Lateral femoral tibial | Patella femoral | Medial femoral tibial | ||||
“r” | “P” | “r” | “P” | “r” | “P” | |
Lateral femoral tibial | 0.990 | <0.001 | ||||
Patellofemoral | 0.990 | <0.001 | ||||
Medial femoral tibial | 0.977 | <0.001 |
MRI: Magnetic resonance imaging, DECT: Dual-energy computed tomography, CT: Computed tomography
S. No. | Variables | Total (n=35) | No diff. (n=4) | 0.10–0.20 mm (n=31) | Statistical significance | |||
---|---|---|---|---|---|---|---|---|
No. | % | No. | % | c2 | “P” | |||
1. | Age | |||||||
≤45 years | 14 | 1 | 7.1 | 13 | 92.9 | 0.423 | 0.515 | |
≥46 years | 21 | 3 | 14.3 | 18 | 85.7 | |||
2. | Gender | |||||||
Female | 13 | 0 | 0.0 | 13 | 100.0 | 2.669 | 0.102 | |
Male | 22 | 4 | 18.2 | 18 | 81.8 | |||
3. | Pain duration | |||||||
1–15 days | 17 | 4 | 23.0 | 13 | 76.5 | 4.782 | 0.092 | |
16–30 days | 14 | 0 | 0.0 | 14 | 100.0 | |||
>30 days | 4 | 0 | 0.0 | 4 | 100.0 | |||
4. | Obesity | |||||||
Non-obese | 23 | 2 | 8.7 | 21 | 91.3 | 0.495 | 0.482 | |
Obese | 12 | 2 | 16.7 | 10 | 83.3 | |||
5. | Diabetes | |||||||
Non-diabetic | 22 | 4 | 18.2 | 18 | 81.8 | 2.669 | 0.102 | |
Diabetic | 13 | 0 | 0.0 | 13 | 100.0 | |||
6. | Hypertension | |||||||
Normotensive | 26 | 2 | 7.7 | 24 | 92.3 | 1.394 | 0.238 | |
Hypertensive | 9 | 2 | 22.2 | 7 | 77.8 |
S. No. | Variables | Total (n=35) | No diff. (n=4) | 0.10–0.20 mm (n=31) | Statistical significance | |||
---|---|---|---|---|---|---|---|---|
No. | % | No. | % | c2 | “P” | |||
1. | Age | |||||||
≤45 years | 14 | 1 | 7.1 | 13 | 92.9 | 0.423 | 0.515 | |
≥46 years | 21 | 3 | 14.3 | 18 | 85.7 | |||
2. | Gender | |||||||
Female | 13 | 0 | 0.0 | 13 | 100.0 | 2.669 | 0.102 | |
Male | 22 | 4 | 18.2 | 18 | 81.8 | |||
3. | Pain duration | |||||||
1–15 days | 17 | 4 | 23.0 | 13 | 76.5 | 4.782 | 0.092 | |
16–30 days | 14 | 0 | 0.0 | 14 | 100.0 | |||
>30 days | 4 | 0 | 0.0 | 4 | 100.0 | |||
4. | Obesity | |||||||
Non-obese | 23 | 2 | 8.7 | 21 | 91.3 | 0.495 | 0.482 | |
Obese | 12 | 2 | 16.7 | 10 | 83.3 | |||
5. | Diabetes | |||||||
Non-diabetic | 22 | 4 | 18.2 | 18 | 81.8 | 2.669 | 0.102 | |
Diabetic | 13 | 0 | 0.0 | 13 | 100.0 | |||
6. | Hypertension | |||||||
Normotensive | 26 | 2 | 7.7 | 24 | 92.3 | 1.394 | 0.238 | |
Hypertensive | 9 | 2 | 22.2 | 7 | 77.8 |
S. No. | Variables | No diff. (n=13) | 0.10–0.20 mm (n=17) | >0.20 mm (n=5) | ||||
---|---|---|---|---|---|---|---|---|
1. | Age | |||||||
≤45 years | 14 | 6 | 42.9 | 8 | 57.1 | 0 | 0.0 | |
≥46 years | 21 | 7 | 33.3 | 9 | 42.9 | 5 | 23.8 | |
c2=3.891;P=0.143 | ||||||||
2. | Gender | |||||||
Female | 13 | 4 | 30.8 | 7 | 53.8 | 2 | 15.4 | |
Male | 22 | 9 | 40.9 | 10 | 45.5 | 3 | 13.6 | |
c2=0.362;P=0.834 | ||||||||
3. | Pain duration | |||||||
1–15 days | 17 | 9 | 52.9 | 7 | 41.2 | 1 | 5.9 | |
16–30 days | 14 | 4 | 28.6 | 8 | 57.1 | 2 | 14.3 | |
>30 days | 4 | 0 | 0.0 | 2 | 50.0 | 2 | 50.0 | |
c2=7.722;P=0.102 | ||||||||
4. | Obesity | |||||||
Non-obese | 23 | 10 | 43.5 | 13 | 56.5 | 0 | 0.0 | |
Obese | 12 | 3 | 25.0 | 4 | 33.3 | 5 | 41.7 | |
c2=11.181;P=0.004 | ||||||||
5. | Diabetes | |||||||
Non-diabetic | 22 | 11 | 50.0 | 9 | 40.9 | 2 | 9.1 | |
Diabetic | 13 | 2 | 15.4 | 8 | 61.5 | 3 | 23.1 | |
c2=4.471;P=0.107 | ||||||||
6. | Hypertension | |||||||
Normotens | 26 | 11 | 42.3 | 11 | 42.3 | 4 | 15.4 | |
Hypertense | 9 | 2 | 22.2 | 6 | 66.7 | 1 | 11.1 | |
c2=1.628;P=0.443 |
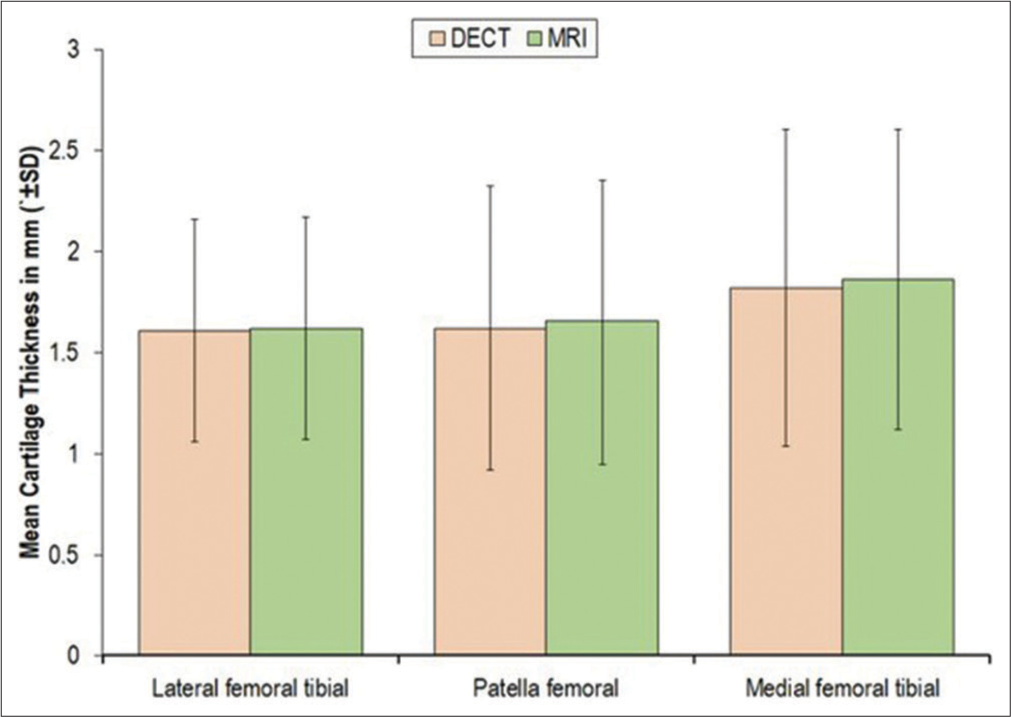
- Measurement of knee cartilage thickness by dual-energy computed tomography and magnetic resonance imaging. DECT: Dual-energy computed tomography, SD: Standard deviation.
![Level of difference of measurements of knee cartilage thickness by dual-energy computed tomography (DECT) (as compared to magnetic resonance imaging [MRI]). The study found no statistically significant differences in cartilage thickness measurements between DECT and MRI based on factors such as age, gender, duration of pain, comorbidities, except for femoral tibial cartilage thickness and obesity, which showed a significant association.](/content/107/2024/6/2/img/IJMSR-6-095-g006.png)
- Level of difference of measurements of knee cartilage thickness by dual-energy computed tomography (DECT) (as compared to magnetic resonance imaging [MRI]). The study found no statistically significant differences in cartilage thickness measurements between DECT and MRI based on factors such as age, gender, duration of pain, comorbidities, except for femoral tibial cartilage thickness and obesity, which showed a significant association.
Overall, the findings suggest that DECT measurements of cartilage thickness are comparable to those obtained with MRI, indicating the effectiveness of DECT in this context.
DISCUSSION
The knee is a vulnerable joint that undergoes significant stress during daily activities, including lifting, kneeling, and high-impact activities such as running and aerobics. It is composed of the tibia, femur, and patella, each of which is protected by a layer of cartilage that absorbs shock and safeguards the knee.[16]
Knee pain is a common issue affecting people of all ages, particularly those who are overweight or engage in sports, farming, or construction work involving repetitive knee stress. Knee pain impacts people of all ages and disrupts their daily routines. While mild knee pain may be managed with muscle relaxant ointments, sprays, knee braces, and physical therapy, severe symptoms such as fever, knee swelling, decreased knee flexion and extension, and the inability to bear weight require immediate medical attention.
To identify the precise cause of knee pain and determine the appropriate management, a thorough medical examination is necessary. Risk factors such as family history of OA, trauma, occupation, overweight, smoking, and others need to be assessed. Laboratory examinations, including joint fluid culture, and radiological tests such as X-rays, CT scans, and MRIs of the knee, are essential steps in this process.
Pain in the knee is often linked to cartilage damage. Healthy cartilage lacks pain receptors, but as it erodes due to various factors, it can expose nerve fibers, potentially becoming a source of pain.[17] In addition, chondrocytes, which are cartilage cells, produce nerve growth factor, which can contribute to pain.[18] Other tissues in the joint, such as the synovium and bone, contain numerous nerve fibers and can also be sources of pain. Cartilage loss can trigger pain directly or indirectly.[19]
Conventionally, assessments of cartilage loss were based on joint space measurements using X-rays, which served as a proxy for cartilage loss. However, these studies could not establish a direct link between joint space loss and worsening pain. In later stages, irreversible changes, like osteocyte formation, were observed.[20] More recently, MRI has become a valuable tool for non-invasive assessment of articular cartilage thickness.[21] Conventional CT arthrography has been used to evaluate surface irregularities in cartilage but is limited in its invasiveness and evaluation capabilities.[22]
Cross-sectional MRI data have shown that less cartilage tends to be associated with more pain.[23] Thus, MRI has become the gold standard for assessing knee cartilage thickness.[24] However, it is limited by factors such as accessibility, long scanning times, and high costs.
The present study was conducted to determine the accuracy of DECT in assessing cartilage thickness in the knee. To achieve this, 35 patients with knee pain requiring medical attention were enrolled in the study.
The study aimed to compare DECT and MRI as diagnostic modalities for assessing cartilage thickness in patients with knee pain. Cartilage thickness was evaluated in the lateral femoral tibial, patella femoral, and medial femoral tibial regions of the knee. The study found that the cartilage thickness measurements obtained with DECT and MRI were in almost perfect agreement, with no significant differences at all regions assessed.
The study’s participants had an age range of 40–57 years, with a mean age of 47.26 years. The majority were aged over 45 years, and the study population was predominantly male (62.9%). The duration of severe knee pain varied from 1 to 90 days, and a history OA was observed in 22.9% and 31.4% of the patients, respectively. Only 34.3% of the participants were classified as obese.
When comparing this study to existing literature, it is evident that there is variation in study design, objectives, study populations, imaging modalities used, and the specific anatomical sites where cartilage thickness is assessed.
For instance, Shah et al.[25] assessed cartilage thickness in patients with KL grade 0–1 and found differences in thickness compared to the present study. Bedewi et al.[26] assessed cartilage thickness using ultrasonography in a younger and healthier population, with different measurement sites. Si et al.[27] reported different anatomical sites for cartilage thickness assessment, but the findings were not directly comparable. Therefore, there is variance in findings based on the specific characteristics of each study.
Several comparative studies of imaging modalities have demonstrated the effectiveness of DECT for various musculoskeletal pathologies, including knee injuries and bone edema. These studies have found DECT to be highly sensitive and specific, with diagnostic accuracy comparable to or exceeding MRI.
However, this study has certain limitations such as small sample size, which may limit the generalizability of the results, the potential for selection bias in patients undergoing both CT and MRI and the lack of long-term follow-up data to assess the progression of cartilage degeneration. Due to the relatively small sample size, our study may not have sufficient statistical power to detect significant differences in knee articular cartilage thickness between healthy and OA groups using DECT and MRI. Future studies with larger samples are necessary to confirm and generalize our findings.
In summary, the results of the present study suggest that DECT has the potential to replace MRI for assessing knee cartilage thickness and qualitative knee abnormality assessments. While MRI is currently considered the gold standard, it has limitations related to cost, accessibility, and patient convenience. DECT offers a promising alternative that provides accurate and reliable results, making it a valuable tool in the assessment of knee conditions.
CONCLUSION
This study aimed to assess the cartilage thickness in the knee joints of patients with knee pain using DECT and correlate it with MRI. The study included 35 patients, all aged 40 years or older, with a mean age of 47.26 ± 4.82 years, and a majority of them were male. The duration of knee pain ranged from 1 to 90 days, with an average duration of 19.91 ± 18.94 days. Notably, 51.4% of the patients experienced knee pain for more than 15 days, and a fraction had a history of knee injury (22.9%) or a family history of OA (31.4%). In addition, the study reported the incidence of hypertension (25.7%), diabetes mellitus (37.1%), and obesity (34.3%).
The study found that knee cartilage thickness measurements using DECT and MRI were quite similar across different regions (lateral femoral tibial, patellofemoral, and medial femoral tibial), with DECT values ranging from 1.61 to 1.82 mm and MRI values from 1.62 to 1.86 mm. The differences between the two modalities were minor, with variations between 0.88% and 2.39%, and were not statistically significant. Most patients showed no meaningful differences, although in some cases, differences >0.20 mm were noted. The correlation between DECT and MRI measurements was nearly perfect in all regions. Factors such as age, gender, pain duration, and comorbidities such as diabetes and hypertension did not affect the differences in measurements. However, mild discrepancies in medial femoral tibial cartilage thickness were more frequently observed in obese patients compared to non-obese individuals.
The study concluded that DECT measurements of knee cartilage thickness were as reliable as those obtained through MRI. DECT offers unique insights into cartilage composition, including the detection of early mineralization and calcifications, which are not visible on MRI, which could be useful in detecting early OA or in cases of suspected chondrocalcinosis. DECT complements the MRI findings to provide a more comprehensive evaluation of knee pathology. This suggests that DECT can be a valuable tool for knee pain patients, particularly in low-resource healthcare facilities where MRI may not be available or when MRI is contraindicated.
Ethical approval
The research/study approved by the Institutional Review Board at Era’s Lucknow Medical College and Hospital, Lucknow, number R Cell EC/2021/32, dated 17th February 2021.
Declaration of patient consent
The authors certify that they have obtained all appropriate patient consent.
Conflicts of interest
There are no conflicts of interest.
Use of artificial intelligence (AI)-assisted technology for manuscript preparation
The authors confirm that there was no use of artificial intelligence (AI)-assisted technology for assisting in the writing or editing of the manuscript and no images were manipulated using AI.
Financial support and sponsorship
Nil.
References
- Prevalence of knee pain and its influence on quality of life and physical function in the Korean elderly population: A community-based cross-sectional study. J Korean Med Sci. 2011;26:1140-6.
- [CrossRef] [PubMed] [Google Scholar]
- Burden of reduced work productivity among people with chronic knee pain: A systematic review. Occup Environ Med. 2014;71:651-9.
- [CrossRef] [PubMed] [Google Scholar]
- The epidemiology of chronic pain in the community. Lancet. 1999;354:1248-52.
- [CrossRef] [PubMed] [Google Scholar]
- Obesity profiles with knee osteoarthritis: Correlation with pain, disability, disease progression. Obesity (Silver Spring). 2007;15:1867-76.
- [CrossRef] [PubMed] [Google Scholar]
- Increasing prevalence of knee pain and symptomatic knee osteoarthritis: Survey and cohort data. Ann Intern Med. 2011;155:725-32.
- [CrossRef] [PubMed] [Google Scholar]
- Non-operative management of osteoarthritis of the knee joint. J Clin Orthop Trauma. 2016;7:170-6.
- [CrossRef] [PubMed] [Google Scholar]
- Electromechanical properties of human osteoarthritic and asymptomatic articular cartilage are sensitive and early detectors of degeneration. Osteoarthritis Cartilage. 2018;26:405-13.
- [CrossRef] [PubMed] [Google Scholar]
- Restoration of injured or degenerated articular cartilage. J Am Acad Orthop Surg. 1994;2:192-201.
- [CrossRef] [PubMed] [Google Scholar]
- What you need to know about cartilage damage. 2019. Medical News Today. Available from: https://www.medicalnewstoday.com/articles/171780 [Last accessed on 2024 Sep 25]
- [Google Scholar]
- The relationship between cartilage loss on magnetic resonance imaging and radiographic progression in men and women with knee osteoarthritis. Arthritis Rheum. 2005;52:3152-9.
- [CrossRef] [PubMed] [Google Scholar]
- Imaging of immature articular cartilage using ultrasound backscatter microscopy at 50 MHz. J Orthop Res. 1995;13:963-70.
- [CrossRef] [PubMed] [Google Scholar]
- Bone marrow lesions in knee osteoarthritis: Regional differences in tibial subchondral bone microstructure and their association with cartilage degeneration. Osteoarthritis Cartilage. 2019;27:1653-62.
- [CrossRef] [PubMed] [Google Scholar]
- First performance evaluation of a dual-source CT (DSCT) system. Eur Radiol. 2006;16:256-68.
- [CrossRef] [PubMed] [Google Scholar]
- The pilot study of dual-energy CT gemstone spectral imaging on the image quality of hand tendons. Clin Imaging. 2013;37:930-3.
- [CrossRef] [PubMed] [Google Scholar]
- Virtual non-calcium dual-energy CT: Clinical applications. Eur Radiol Exp. 2021;5:38.
- [CrossRef] [PubMed] [Google Scholar]
- Knee pain and problems. Available from: https://www.hopkinsmedicine.org/health/conditions-and-diseases/knee-pain-and-problems [Last accessed on 2024 Sep 25]
- [Google Scholar]
- The association of bone marrow lesions with pain in knee osteoarthritis. Ann Intern Med. 2001;134:541-9.
- [CrossRef] [PubMed] [Google Scholar]
- TGF-β is a potent inducer of nerve growth factor in articular cartilage via the ALK5-Smad2/3 pathway. Potential role in OA related pain? Osteoarthritis Cartilage. 2015;23:478-86.
- [CrossRef] [PubMed] [Google Scholar]
- Does cartilage loss cause pain in osteoarthritis and if so, how much? Ann Rheum Dis. 2020;79:1105-10.
- [CrossRef] [PubMed] [Google Scholar]
- How does tibial cartilage volume relate to symptoms in subjects with knee osteoarthritis? Ann Rheum Dis. 2004;63:264-8.
- [CrossRef] [PubMed] [Google Scholar]
- Quantification of articular cartilage in the knee with pulsed saturation transfer subtraction and fat-suppressed MR imaging: Optimization and validation. Radiology. 1994;192:485-91.
- [CrossRef] [PubMed] [Google Scholar]
- Knee arthrography. Evolution and current status. Radiol Clin North Am. 1998;36:703-28.
- [CrossRef] [PubMed] [Google Scholar]
- Association of magnetic resonance imaging-based knee cartilage T2 measurements and focal knee lesions with knee pain: Data from the osteoarthritis initiative. Arthritis Care Res (Hoboken). 2012;64:248-55.
- [CrossRef] [PubMed] [Google Scholar]
- Determination of 3D cartilage thickness data from MR imaging: Computational method and reproducibility in the living. Magn Reson Med. 1999;41:529-36.
- [CrossRef] [Google Scholar]
- Variation in the thickness of knee cartilage. The use of a novel machine learning algorithm for cartilage segmentation of magnetic resonance images. J Arthroplasty. 2019;34:2210-5.
- [CrossRef] [PubMed] [Google Scholar]
- Sonographic assessment of femoral cartilage thickness in healthy adults. J Int Med Res. 2020;48:300060520948754.
- [CrossRef] [PubMed] [Google Scholar]
- Knee cartilage thickness differs alongside ages: A 3-T magnetic resonance research upon 2,481 subjects via deep learning. Front Med (Lausanne). 2021;7:600049.
- [CrossRef] [PubMed] [Google Scholar]